High Energy Density Physics and Planetary Physics
Overview
Over the past few decades, planetary science has seen a tremendous horizon widening. Nowadays, planetary science stretches from the detailed investigation of our Solar system planets to the detection and characterization of thousands of planets around other stars. These so-called exoplanets span orders of magnitude in planet mass (~1 Earth mass to ~20 Jupiter masses) with some overlap to the brown dwarf regime, planet radius (~0.1 Earth radii to about 2 Jupiter radii), and orbital distances (~0.01 AU to ~100 AU). Therefore, if we seek to understand their physical nature and compare them to the planet we inhabit (Earth) and its farther out neighbors (like Jupiter), knowledge of material properties across wide ranges in temperature, pressure, and composition are required.
In this Working Group we investigate the internal structure, thermal evolution, and physical make-up of all classes of planets and of brown dwarfs.
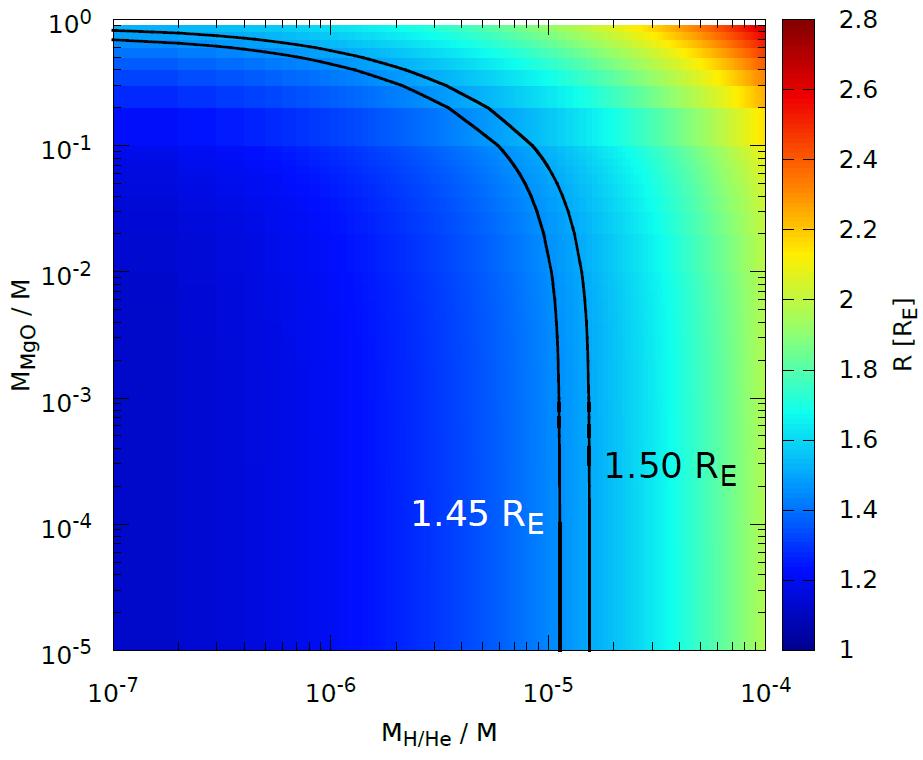
Planets of sizes intermediate to Earth and Neptune (super-Earths/mini-Neptune) appear to be an especially common class of planets. Given measured radius and mass, we model their internal structure in order to derive their possible bulk composition in terms of iron-to-rock ratio, ice-to-rock ratio, or their atmospheric thickness. As the tidal response of a planet is particularly sensitive to the composition, we also explore how the tidal Love number further constraints the internal structure.
Part of this work is performed within the DFG-funded Research Unit FOR 2440/1.
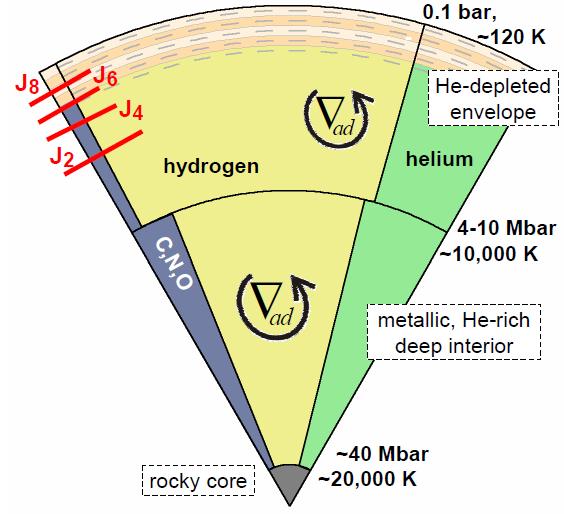
The biggest planet in our Solar system, Jupiter, stands perpetually in the center of our research efforts. We use Jupiter to probe equations of state for its main constituents hydrogen and helium in the warm dense matter regime of several Mbar pressures and ~1000 to ~20,000 K, where ions behave strongly coupled and electrons feel their fermion nature. There, lab experiments become challenging and expensive while Jupiter's interior just provides those conditions.
In turn, we also aim to understand the internal structure of Jupiter and other gas giant planets. For that purpose we apply our own data base for material properties for H/He and minor constituents and construct models for the planetary internal structure and thermal evolution constrained by observational data. Thanks to various past (Pioneer 10,11, Voyager 1,2) and current (Cassini, Juno) missions, a wealth of accurate observational constraints on Jupiter and Saturn exist. This is especially true for the gravity field in terms of the low-order gravitational harmonics J2 to J4, which probe the density distribution down to Megabar pressure levels. In fact, the reduced uncertainties in the acquired observational data and in the material properties nowadays challenge the common perception of a homogeneous fluid envelope + core structure for gas giant planets. Therefore, we are developing new Jupiter and Saturn models. The actual work here consists of computer code development based on well-known techniques. Scientifically, we pursue the questions of
- the size of the rock-iron core in gas giant planets (does Jupiter have a core?),
- the internal distribution of heavy elements (are gas giants fully homogeneous? why not?)
- the properties of the magnetic field generating regions (where have Saturn's high-order field components gone?)
- the possibility of H/He phase separation in Jupiter and Saturn and the influence on the observable luminosity (is Saturn's brightness caused by gravitational settling of helium droplets?)
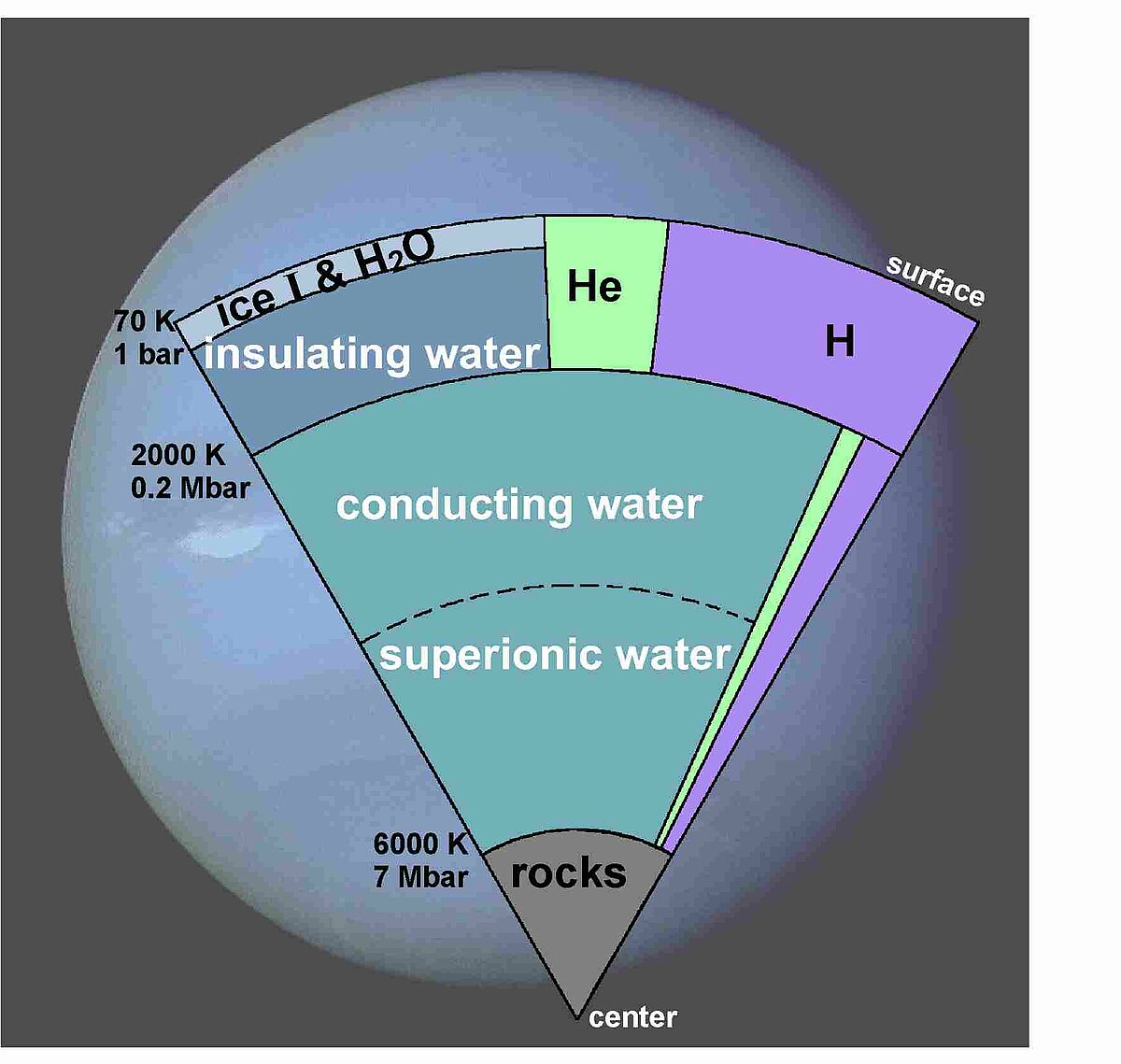
Uranus and Neptune are often labeled ice giants since planet interior models are consistent with a composition of about 85% water and their low surface temperatures of -200 degree Celsius suggest ice-forming components (water, ammonia, methane) to be frozen. However, interior models as developed also in this Working Group suggest the outer ~20% to consist of mostly H/He rather than water, in which case the envelope remains fluid and temperatures rise steeply --preventing the entire planet from freezing. Moreover, it is unclear at present if Neptune-sized planets close to their parent star as found in many extrasolar systems are water-rich- or if they may be gas and rock dominated, unlike any planet in the solar system.
In this working Group we apply equations of state and material properties of water, methane, and ammonia to Uranus and Neptune and investigate the possible internal structure and thermal evolution of ice giants as a class of planets. So far, all models for Uranus and Neptune fail in some respect or the other to explain the relevant observational constraints, foremost the faintness of Uranus, the brightness of Neptune, and both planets' unusual magnetic field geometries. We address the questions
- of the temperature profile in Uranus and Neptune and the corresponding possible phases of water/ammonia/methane
- is the deep interior super-ionic and how would that influence the magnetic field ?
- is there a thermal boundary somewhere in the planet and can that explain the luminosities ?
- is Uranus faint because the interior is cold, or is Uranus faint because the heat is trapped in warm core?
- is the deep interior fully convective or is the dynamo region confined to a thin shell of fluid, conducting water ?
- can the tidal Love number, if measured, help to distinguish between a H/He + rock and a water-rich composition ?
- are the constituents nicely miscible with each other or may carbon prefer to separate from water, forming perhaps a diamond layer ?
These questions are partly pursued within the Research Unit FOR 2440/1.